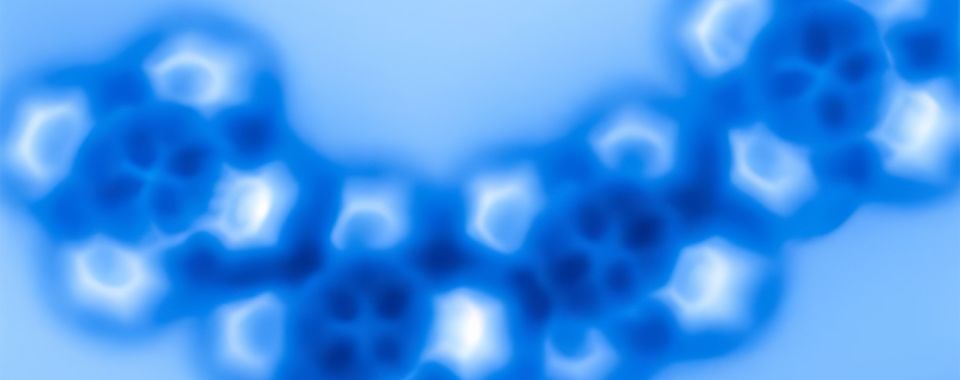
Electron orbits shown in an image made with an atomic force microscope at the Princeton Materials Institute. Images by Princeton University
By John Sullivan
July 14, 2023
Orchestrating an array of advanced microscopes, researchers at Princeton and the University of Texas have created images of molecules with such clarity that it is possible to distinguish iron from cobalt by the orbital shapes of electrons buzzing around them.
Chemists have long used abstract shapes to describe electron orbitals in theory, which are crucial to how atoms and molecules behave. However, this groundbreaking study marks the first direct observation of these orbital shapes, rather than inferring them based on chemical reactions.
“People have predicted certain orbital structures, but they have never seen them,” said Nan Yao, a lead researcher and the director of the Imaging and Analysis Center at the Princeton Materials Institute. Yao, a professor of the practice at Princeton, said the research team was surprised when they first looked at the images and were able to distinguish one element from another by the orbital shapes alone.
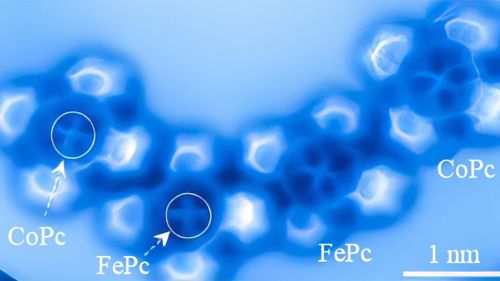
No longer are electron orbitals the simple spheres and dumbbells of chemistry textbooks. Instead, these atoms revealed distinct features which allowed the researchers to tell cobalt from iron in the same way a birdwatcher could tell a blue jay from a bluebird. In the image, the iron atom has a squarish shape, while cobalt is brighter with more distinct lobes in its electron orbitals.
The researchers, reporting in the journal Nature Communications, found it was possible to distinguish electron orbital structures of atoms within a molecule using an atomic force microscope. The microscope operates by running an incredibly sharp probe, in this case a single carbon monoxide molecule, over a target molecule’s surface. The probe responds to quantum forces from the target, sending data to the microscope’s computer and displaying a direct image. Researchers then used supercomputers at the University of Texas to perform the calculations that allowed them to create simulated images that show the same orbital patterns of electrons within the molecules.
“It is so amazing to see this for the first time, actually to see it,” said Craig Arnold, one of the project’s researchers.
Arnold, the Susan Dod Brown Professor of Mechanical and Aerospace Engineering and vice dean for innovation at Princeton, said that understanding the properties of electron orbitals is a foundation of physics and chemistry. He said that science has unlocked many properties through measurement and experiments, but observing molecules directly provides a new way of comprehending their behavior. This knowledge can be used by scientists to gain deeper understanding, improve the design of new materials, and shape chemical reactions.
Beyond the technical importance, Arnold said, the experiment represents science at its most basic level, the drive to “observe something that could not be observed before.”
“It is absolutely remarkable,” he said.
Yao said the researchers chose iron and cobalt because they have very similar properties and are next to each other on the periodic table.
“The difference of a single electron between these two elements provides us with an opportunity to delve deeper into the distinctions arising from subtle variations in electron occupancy within electron orbitals,” Yao said. “Iron and cobalt are so alike, differing by only one electron, but we can distinguish them.”
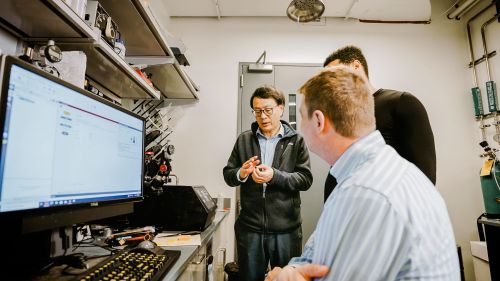
The team conducted the microscopy in Princeton’s Imaging and Analysis Center, which features a suite of the world’s most advanced equipment. The center is built deep in the New Jersey bedrock to prevent vibrations from disturbing the experiments. At one point, Yao recalled, the installation of some of the equipment was adjusted to avoid potential vibration from an elevator in a building down the street. The vibrations in the center are less than a micron per second, about 1/70th the thickness of a human hair. Still, many experiments are run at night to minimize shaking.
Even under these conditions, setting up the experiment was challenging. It is impossible to point a microscope at a particular isolated atom and start taking images. Atoms and molecules skitter about, extremely sensitive to changes in their environment. The researchers had to develop a molecular scaffold to pin their target down without affecting the instruments’ ability to take an image.
Yao chose the chemical phthalocyanine as that scaffold. In a phthalocyanine molecule, rings of carbon and nitrogen atoms join in a four-leaf clover shape, with a single metal atom stuck in the center. Yao made samples of iron phthalocyanine and cobalt phthalocyanine and deposited them sequentially on an ultra-clean copper plate. A weak attraction between the copper and the metals gently pinned the molecules to the plate.
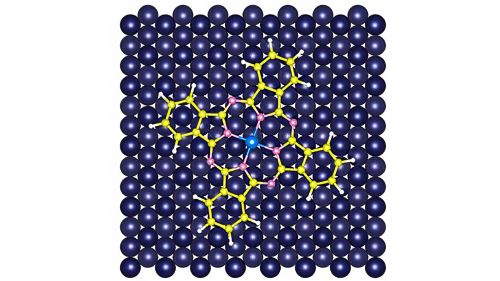
The researchers then used two types of specialized microscopes to create images of the electron clouds. Researchers used a scanning tunneling microscope to gather initial information about the molecules. They used an atomic force microscope for high-resolution imaging. Operating at temperatures just slightly above absolute zero, the microscope’s probe — a single carbon monoxide molecule — moved systematically over the phthalocyanine molecules.
“It’s like touching your desk with your eyes closed to recreate the topological surface of the desk,” said Pengcheng Chen, an associate research scholar at the Princeton Materials Institute who helped supervise the procedure. “Each time you touch the surface, you gather information of each pixel.”
The microscope collects data from a variety of forces. The readings are made at incredibly close range, about 0.3 nanometers. At that distance, the electron orbitals of the two atoms, the probe, and its target, begin to overlap. Because the orbitals repel each other, this generates a force, called the Pauli repulsion force, which the microscope reads.
“The Pauli repulsion is the dominant force contributing to our reading,” Chen said.
James Chelikowsky, the W.A. “Tex” Moncrief, Jr. Chair of Computational Materials at the University of Texas, and Dingxin Fan analyzed the data from the microscopes using the Stampede2 supercomputer at the Texas Advanced Computing Center. Fan, now a postdoctoral researcher at Princeton, was advised by Chelikowsky while pursuing his doctorate at Texas. Chelikowsky said the forces involved were incredibly small but were distinguishable using the computer.
“When we did the calculations, you can see the differences,” he said. “They are subtle.”
The experiment followed 2021 work in which the research team used the atomic force microscope to observe the breaking of a single chemical bond. Yao said the researchers would like to expand their efforts to other atoms, as well as further efforts to explore new aspects of atomic structure.
“Science is driven by experimental observations,” Yao said. “We don’t always know what we will find until someone does the experiments.”
The article, “Observation of Electron Orbital Signatures of Single Atoms within Metal-Phthalocyanines using Atomic Force Microscopy,” was published March 16 in the journal Nature Communications. Besides Yao, Arnold, Chen, Chelikowsky, and Fan, authors include Annabella Selloni, the David B. Jones Professor of Chemistry at Princeton University; Emily Carter, the Gerhardt R. Andlinger Professor in Energy and the Environment; Yunlong Zhang and Adam Gross, of ExxonMobil Technology and Engineering Company. Support for the project was provided in part by the National Science Foundation-MRSEC program, the Welch Foundation, ExxonMobil through its membership in the Princeton E-ffiliates Partnership of the Andlinger Center for Energy and the Environment, the Innovation Award from the J. Insley Blair-Pyne Fund, and the Evin-Catalysis Initiative Award at Princeton.