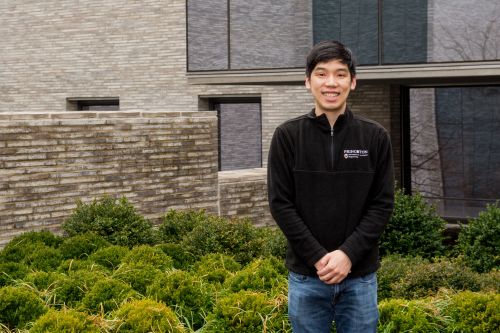
April 2021
Timothy Chen grew up in the small suburb of Los Altos, Calif., a 10-minute drive from Apple’s headquarters on the western edge of Silicon Valley. The movie, “Jobs,” about the company’s iconic founder, was partially filmed at Steve Job’s childhood home, one street behind Tim’s backyard.
As Tim began to explore college options, his family encouraged him to pursue computer science to take advantage of the area’s high-tech career opportunities. But programming as a career didn’t interest him much.
As a team member in the FIRST Robotics Competition in high school, however, he was intrigued with the mechanics of robot building. Tim would go on to earn his undergraduate degree in mechanical engineering at the University of Illinois at Urbana-Champaign. That’s where he took an introductory course in thermodynamics and power engineering. The path into the world of energy technology had found him.
"I realized I was learning about how power plants work, and it was interesting to see how all the different components tie together for energy generation and conversion,” Tim says.
His desire to delve more deeply into energy research brought him to the Department of Mechanical and Aerospace Engineering (MAE) at Princeton University.
Now a fifth-year graduate student in Professor Yiguang Ju’s lab, Tim uses laser diagnostics to study the potential for plasma-assisted conversion of carbon dioxide, methane and other gases into sustainable chemicals and synthetic fuels. Plasma is ionized gas that is electrically conductive.
Before Princeton, Tim had never worked with lasers. But he was drawn to the “magical” aspects of nonequilibrium plasma. He was also interested in how lasers are enabling researchers to learn more about how this fourth state of matter could be used for more efficient combustion, as well as to turn greenhouse gasses like carbon dioxide and methane into sustainable chemicals in a process called dry reforming.
“Nonequilibrium plasma is like magic, in the sense that it allows you to do things you normally wouldn't be able to do,” Tim says.
Unlike the fully ionized plasma of the sun where the temperature is so hot that the gas molecules break down into a soup of disconnected subatomic particles, nonequilibrium, or cold, plasmas are partially, or weakly, ionized. Temperatures of the highly energetic electrons can reach 10,000 Kelvin (about 17,540 degrees Fahrenheit), but the rest of the gas is not much above room temperature.
These different temperature levels create distinct chemical pathways, as the energetic electrons collide into ionized and stable molecules. Such pathways could be used to enhance chemical reactions in other materials. However, there is little understanding about exactly how nonequilibrium plasma interacts with chemical elements to improve efficiency.
As a first-year graduate student, Tim conducted research that centered around understanding how the energy of free electrons in weakly ionized argon or helium influences chemical behavior.
Accurate measurements of electron temperature and density are critical in investigating these plasmas. But obtaining the high-resolution measurements needed to study temperature and density simultaneously to gain new insights meant that Tim needed access to a rare and expensive Thomson scattering device, which Princeton did not have at the time. So, he built one.
To help him achieve this monumental task, his co-advisor, MAE Assistant Professor Egemen Kolemen, sent him to the Dutch Institute for Fundamental Energy Research in the Netherlands, one of the few institutions that had such a device. Tim spent a week there to learn the basic design parameters.
It would take him nearly a year to build and test his device, which he cobbled together using mostly off-the-shelf parts. He designed the rest of the components, which were produced by Princeton’s machine shop technicians.
“Going from zero to building something that enabled me to measure something as elusive as these electrons was really amazing, and I finally felt a sense of accomplishment,” Tim says.
One goal of Tim’s research is to use plasma to turn natural gas, mostly consisting of methane, directly into methanol to reduce flaring, the process of burning off gas in a controlled manner. Gas leakage during oil extraction and processing on rigs is common. In this case, it’s uneconomical to capture, store and transport in highly pressurized pipelines what is largely considered methane “waste.”
Methanol, which is used as a feedstock for many commodity chemicals, such as formaldehyde and acetic acid, can be easily stored onsite and transported using existing oil pipes. Plasma might even be able to help make methanol a more viable fuel.
Tim has since developed novel techniques to measure other plasma characteristics, such as the electric field.
“If we can measure the local electric field in these cold plasmas, then we can better understand how the plasma pulsing affects the electrons in the various chemistries we are trying to influence,” Prof. Kolemen says. “Tim developed a SEE-FISH method, which enhances our ability to obtain these electric field measurements.”
SEE-FISH stands for Split Excitation Electric Field Induced Second Harmonic generation. It’s an extension of a method, developed at Princeton, that uses a single high-intensity laser to measure the electric field. The challenge was having to use the laser in repeated experiments and then piecing together all the measurements to see how the plasma properties evolve over time.
To address this issue, Tim enhanced the technique by splitting the laser into multiple parts and then time delaying them. This enables the use of a single plasma discharge to more effectively study how the electric field changes over time.
In studying molecules in plasma, Tim also has made notable contributions at Sandia National Laboratories, where he collaborated with a research team for five months under the U.S. Department of Energy’s Office of Science Graduate Student Research Fellowship program.
Using Sandia’s femtosecond and picosecond lasers, he was instrumental in developing a technique that simultaneously measures molecular rotational and vibrational states with high spatial resolution.
“This new method enables us to get really close to the plasma-surface boundary to learn more about potential interactions with the catalytic surfaces and how that could benefit chemical conversion,” Tim says.
“Tim has made exceptional contributions in time and space resolved laser diagnostics of plasma chemistry,” says Prof. Ju, who also serves as Tim’s co-advisor. “Plasma-aided chemical reforming will be a transformative technology for negative-carbon chemical synthesis, and understanding the plasma chemistry is critical in enabling this technology.”
Tim plans on continuing to probe the promise of plasma, preferably in a government or academic lab. “Electrifying the chemical processing industry is where I see the promise and flexibility of plasma,” he says.
-- Jodi Frank